Biosynthesis of aromatic amino acids
The aromatic amino acids, phenylalanine, tryptophan, and tyrosine, are all made from a common intermediate: chorismic acid. Chorismic acid is made by the condensation of erythrose‐4‐phosphate and phosphoenol pyruvate, followed by dephosphorylation and ring closure, dehydration and reduction to give shikimic acid. Shikimic acid is phosphorylated by ATP and condenses with another phosphoenol pyruvate and is then dephosphorylated to give chorismic acid.

Figure 1
The condensation of phospho‐shikimic acid with phosphoenol pyruvate is catalyzed by the enzyme 3‐enoylpyruvoylhikimate‐5‐phosphate synthase, or EPSP synthase for short. This reaction is specifically inhibited by the herbicide glyphosate.
Glyphosate is toxic to plants and free‐living microorganisms because it inhibits aromatic amino acid biosynthesis. On the other hand, it is extremely nontoxic to humans and animals because humans derive their amino acids from the diet. Additionally, it is broken down in the soil, so it is non‐persistent. The only problem with glyphosate herbicides is that they will kill crop plants as readily as weeds. Recently, genetically engineered crop varieties have been introduced which are resistant to the herbicide, allowing weeds to be killed preferentially.
Once chorismate is produced, it can be converted to either tryptophan, tyrosine, or phenylalanine by distinct pathways. Additionally, many organisms can hydroxylate phenylalanine to tyrosine. This reaction explains why tyrosine isn't an essential amino acid in humans, even though humans can't make any aromatic amino acids from simple precursors.
Pathway determination by biochemical genetics
The use of genetic mutants determined the complex pathways that lead to the amino acids. A mutant is an organism that has a different DNA sequence from its parent(s). Mutant bacteria that require a specific compound for growth are called auxotrophs. The first step in pathway determination is to assemble a large collection of auxotrophic mutants that can't make the compound of interest.
The pathway for the biosynthesis of tryptophan illustrates the overall process. First, the auxotrophic mutants had to be organized into classes that correspond to the biochemical steps in the pathway. Mutant strains were examined for their Ability to excrete compounds that allowed the growth of other mutants. These cross‐feeding experiments were carried out for pairs of mutants. Thus, the compound excreted from one group of mutants, called Group A, was able to support the growth of other mutants, including Group E. On the other hand, Group E mutants could not excrete a compound that allowed Group A mutants to grow. The fact that Group A mutants excreted a product that allowed Group E mutants to grow, but not vice versa, means that the compound excreted by Group A mutants occurs after the compound excreted by Group E mutants (if any). This is a fundamental idea in determining biochemical pathways.
To understand this idea, consider a biochemical pathway to be like a series of entrances to a highway. Normally, one can enter the highway at any on‐ramp and get to one's destination. But what happens if a wreck occurs between entrances A and E? The wreck blocks the traffic, and the unlucky drivers who got on the highway ahead of the wreck (those who got on at E) can't get to their destination. On the other hand, those who have gotten on at A remain free to travel and won't even notice a delay. See Figure 2.

Figure 2
The same logic was used to identify the groups of mutants who allowed the growth of other mutants, or who were able to grow on the compounds excreted by other groups of mutants. In the case of tryptophan, five such complementation groups existed.
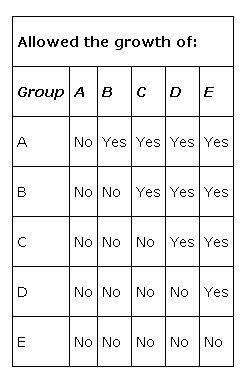
From these data, it should be fairly easy to deduce that complementation group E comes last in the pathway while complementation group A comes first.
This did not establish the pathway completely. The next step was to identify the compounds that are excreted and allow growth of the other mutants. Next, the pathway was established biochemically by identifying and purifying the individual proteins that carry out the steps corresponding to each complementation group, showing that the enzymes behaved kinetically in the way that the other analyses predicted was necessary. These studies showed that the proteins encoded by Groups A and B are part of a complex (called tryptophan synthase). Similarly, the gene products of the D and E groups associate to form the first enzyme, anthranilate synthetase. The overall pathway is as follows:

Figure 3
Feedback inhibition
You can see that biosynthesis of amino acids is a complex, energy‐requiring process. Almost all biosynthetic pathways are regulated so they don't produce too much of the product. This process, feedback inhibition, occurs at the first committed step of the biochemical pathway. In tryptophan biosynthesis, the first committed step is the synthesis of anthranilic acid from chorismate. When excess tryptophan is available, either through biosynthetic reactions or from the environment, it is able to bind to a site on anthranilate synthetase. This binding to the allosteric regulatory site causes the enzyme to be less active. This means that chorismate is diverted to the tyrosine and phenylalanine pathways. Higher levels of tryptophan can partially inhibit the synthesis of chorismate, thus further sparing the cell's energy reserves.
Amino acid catabolism
Excess amino acids are degraded, rather than stored, by almost all biological systems. Seed formation in plants and the synthesis of yolk and proteins in eggs constitute the major exceptions. Thus, a “high‐protein” diet normally provides little benefit. Most healthy individuals need a relatively small amount of dietary protein, unless they are growing children. A typical “Western diet,” with a large meat intake, isn't necessary for health.
The products of amino acid breakdown are of two kinds. Ketone bodies—that is, acetoacetate and hydroxybutyrate—are formed from the catabolism of the branched‐chain amino acids, lysine and some aromatic amino acids. Tricarboxylic acid (TCA) cycle intermediates, including pyruvate and glutamate, are formed from most of the other aliphatic and aromatic amino acids. Amino acids whose metabolisms produce ketone bodies such as acetoacetate are called ketogenic; amino acids whose metabolisms produce TCA cycle intermediates are called glucogenic, because TCA cycle intermediates are substrates for gluconeogenesis. Individual amino acids can be exclusively ketogenic, exclusively glucogenic, or both. Only leucine and lysine are considered to be exclusively ketogenic, and some suspicion remains that they may also give rise to TCA cycle intermediates.
Amino acids are also precursors to biologically important compounds. In animals, removal of the carboxylic acid group from amino acids creates many neurotransmitters.
Dopamine, which is essential for the control of movement, is made from tyrosine (or phenylalanine, because tyrosine is a direct product of phenylalanine metabolism).
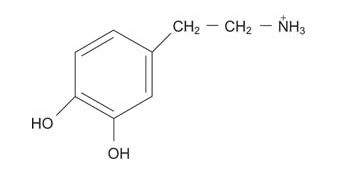
Serotonin, a “sleep‐inducing” transmitter, is made from tryptophan.
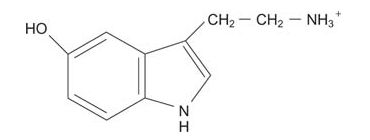
![]()
γ‐aminobutyric acid (GABA) is made from glutamate.
![]()
![]()
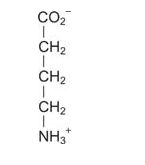
![]()
![]()
Inborn errors of amino acid metabolism
An inability to degrade amino acids causes many genetic diseases in humans. These diseases include phenylketonuria (PKU), which results from an inability to convert phenylalanine to tyrosine. The phenylalanine is instead transaminated to phenylpyruvic acid, which is excreted in the urine, although not fast enough to prevent harm. PKU was formerly a major cause of severe mental retardation. Now, however, public health laboratories screen the urine of every newborn child in the United States for the presence of phenylpyruvate, and place children with the genetic disease on a synthetic low‐phenylalanine diet to prevent neurological damage. ![]()
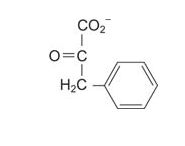
Heme biosynthesis
Hemoglobin and myoglobin carry blood oxygen. Heme is iron bound to a porphyrin ring. Porphyrin biosynthesis begins with δ‐aminolevulinic acid, the condensation product of the amino acid glycine with succinyl‐CoA. The ring is formed by the “head to tail” condensation of two δ‐aminolevulinic acid molecules to form porphobilinogen.
![]()
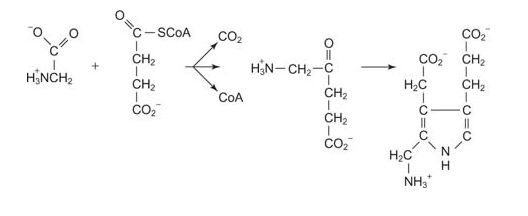
Note the Schiff base formation that forms the 5‐membered ring.
Heme contains four of these rings. Four porphobilinogens condense head to tail to form the first tetrapyrrole species, which is then circularized to form the porphyrin skeleton. Further modifications, followed by Fe(II) addition, lead to heme.

Figure 4
Inborn metabolic diseases that interfere with heme biosynthesis are called porphyrias. Porphyrias have a variety of symptoms. A deficiency in the enzyme responsible for the condensation of porphobilinogen to the 4‐membered ring system leads to a condition called acute intermittent porphyria, which is characterized by occasional episodes of abdominal pain and psychiatric symptoms. Defects in the later enzymes of the pathway lead to an excess accumulation of the uroporphobilinogens in the tissues, where they cause a variety of symptoms, including hairy skin, skeletal abnormalities, light sensitivity, and red urine. Individuals with this disease are still anemic—a condition that can be alleviated somewhat by the heme acquired from drinking blood. This combination of traits sounds like the werewolf and vampire legends of Europe, which may have their base in this rare biochemical disease.
|
|
|
|
|
|